Abstract
There is an opinion that the biological activity of chemical compounds derived from natural raw materials and that of artificially synthesized compounds (analogues of natural substances) may differ. This assumption, however, is still poorly supported by serious scientific research. In our work, we compared a chloroform solution of synthetic juglone (5-hydroxy-1,4-naphthoquinone) and a chloroform extract from a mesocarp of Manchurian walnut (Juglans mandshurica Maxim. (1856)) using the nuclear magnetic resonance (NMR) method to identify possible differences. Research has shown that synthetic and natural juglone are completely identical. This is confirmed by the coincidence of the NMR signals in the spectra of both substances. Differences were found in the content of impurities, although their content is very insignificant and does not exceed 0.1%. With a 15-fold increase in the spectrum of synthetic juglone in the range of 1.5–3.75 ppm, it was seen that the mixture under study contains water in two forms: free and bound (in salts). The presence of these signals allows us to conclude that salts are one of the main impurities in the synthetic juglone sample. The results showed that different forms of 5-hydroxy-1,4-naphthoquinone are present in the synthesized juglone and Manchurian walnut pericarp extract.
Keywords: Naphthoquinone, Synthesis of Organic Compounds, Synthetic Compounds
Introduction
The history of the synthesis of organic compounds dates back almost 200 years. However, the debate of whether natural molecules have fundamental differences from synthetic ones or not has yet to be settled.
Naphthoquinones are important secondary metabolites essential for many biological processes. They are found not only in plants and lichens but also in animals. A vast variety of naphthoquinones that exhibit antitumor (Dyshlovoy, et al., 2019), antimicrobial (Sabutski et al., 2020), antiparasitic, anti-inflammatory, antifungal, and trypanocidal activities (Dayronas & Zilfikarov, 2011) have been discovered to date. There are also several medicinal drugs with the main active agents being naphthoquinones, such as histochrome, vikasol, and atovaquone (also known under the trade name of Merpon) (Hughes et al., 1993). Such a wide range of applications of naphthoquinones raises great interest in these substances both when searching for their sources in nature and when creating synthetic analogues.
Many plant species can synthesize naphthoquinones, whereas normally none of the vertebrate animals (Chordata) synthesize these compounds. Nevertheless, naphthoquinones show high physiological activity having an effect on metabolism, at least, in mammals (Chordata, Mammalia).
It can be assumed that, although vertebrates lack the ability to synthesize naphthoquinones, their cells can interact with them. This is most likely explained as follows: this ability was lost across chordate evolution, but the mechanism of interaction with naphthoquinones in metabolic processes has been partially retained. Mammalians began to compensate for the lack of necessary substances with food or a product of symbiotic bacteria. For example, menaquinone, or vitamin K2 necessary for the activity of a human organism, is synthesized by bacteria in the intestine, but not by human’s glands.
The major group of animals that have retained the ability to produce naphthoquinones are members of the phylum Echinodermata. The phylum comprises almost 7,000 species from five classes.
There is evidence that, besides echinoderms, naphthoquinones are also found in tissues of arthropods:(Eschscholtz, 1822) (Arthropoda, Insecta, Blattodea, Blaberidae). Three naphthoquinones were isolated from the species: 2-ethyl-1,4-benzoquinone; 2-methyl-1,4-benzoquinone; and para-benzoquinone (or 1,4-benzoquinone). Members of the genus have a noteworthy feature: like mammals, they feed their offspring with a secretion similar to milk (Roth & Stay, 1958).
Deriving naphthoquinones from plants is much cheaper than from marine organisms. Representatives of the sea urchins (class Echinoidea, phylum Echinodermata) contain spinochromes, also related to naphthoquinones (Drozdov et al., 2017, 2021). Sea urchins are widely used in traditional Chinese medicine (Hou et al., 2018). Naphthoquinones of the spinochrome class have high biological activity and are promising for the preparation of therapeutic and cosmetic drugs (Brasseur et al., 2017; Fedoreyev et al., 2018; Mishchenko et al., 2020; Vasileva et al., 2021; Vo et al., 2020).
As for the production of naphthoquinones from the latter source, it is associated with several challenges. The major problem is the high cost due to the insufficient amount of natural raw materials. For example, the sand dollar A. Agassiz, 1864, used as a source to extract histochrome (Mishchenko et al., 2004), is often found in its main habitats in large numbers (the waters of the Sea of Japan, Primorsky Krai), but the sizes of the landing of this sea urchin species there cannot meet the needs of industrial production of naphthoquinones. The aquaculture production of echinoderms also faces major problems. For many echinoderms, the crucial factors necessary for successful cultivation are a certain level of water salinity (therefore, marine farms should not be located near river mouths and estuaries), depth, illumination, temperature,., which are sometimes difficult to provide for artificial breeding. For this reason, it is almost impossible to meet the demand for naphthoquinones (at least only in the pharmaceutical industry) using solely natural resources or aquaculture.
Breeding of arthropods such as to isolate various forms of naphthoquinones from them is also a promising approach. To do this, it is necessary to carry out a targeted search for animals that produce these compounds and, however, do not pose difficulties in their artificial breeding.
Therefore, the most promising approach to obtaining naphthoquinones in industrial amounts is their chemical synthesis, which also allows improvement of the quality of natural naphthoquinones by artificially changing their structure. Thus, S.G. Polonik and co-authors from the G.B. Elyakov Pacific Institute of Bioorganic Chemistry, Far Eastern Branch of Russian Academy of Sciences, found that the cytotoxic activity of bromjuglone is higher than that of thioglycosides of juglone (Polonik et al., 1995). Subsequently, they also discovered that acetylated glycosides of juglone exhibit immunomodulatory effects (Polonik et al., 2003).
Problem Statement
Chemicals of the naphthoquinone series are quite easy to synthesize. Thus, juglone (5-hydroxy-1,4-naphthoquinone), first isolated from peels of unripe fruit of the walnut, is synthesized by oxidation of naphthalene oxy derivatives. One of the approaches to the industrial synthesis of 5-hydroxy-1,4-naphthoquinone is described in the book "Juglone and related 1,4-naphthoquinones" by Zhungietu and Vlad (1978). The major advantage of this method is skipping the stage of obtaining a free intermediate product, 1,5-dioxynaphthalene. The product of alkaline melting of the disodium salt of 1,5-naphthalene disulfonic acid is treated with sulfuric acid and then oxidized with chromium salt in the presence of the resulting Glauber salt (Fig. 1).
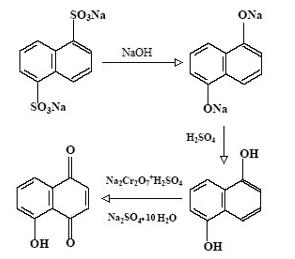
There are two opposing opinions as regards the biological activities of molecules isolated from natural raw materials and synthesized analogues. Some researchers believe that synthetic molecules are completely identical to natural analogues, while others assume that synthesizing a molecule created by nature is impossible and that the biological activity of synthesized molecules is always different from that of molecules created in a living cell. So far, this discussion is rather behind the scenes, without serious scientific research and evidence presented to the scientific community.
Research Questions
The results of comparing the identity of molecules by such methods as chromatography and mass spectrometry are often difficult to interpret, since the molecules, being compared may be of the same molecular weight and show similar retention times on a chromatographic column. Nevertheless, they can have conformational differences, and, therefore, an X-ray fluorescence analysis or NMR spectroscopy is more suitable for such comparisons.
Purpose of the Study
In our work, we studied the synthetically obtained juglone and a chloroform extraction from a mesocarp of Manchurian walnut ( Maxim. (1856)) to understand whether there are differences between natural and synthetic compounds of juglone (5-hydroxy-1,4-naphthoquinone) and to determine what they are.
Research Methods
The synthetic juglone was dissolved in 600 µL of deuterated chloroform (CDCl3) for automatic adjustment of the uniformity of magnetic field along the deuterium channel. The Manchurian walnut mesocarp was crushed and extracted with chloroform; the extract was then dried under airflow and dissolved in the deuterated chloroform. Then the resulting mixtures were supplemented with tetramethylsilane (SiC4H12) and analyzed on a DRX-5 NMR spectrometer (Bruker) with a resonant frequency of 300 MHz at a temperature of 36°C.
The data obtained by the proton 1-D spectroscopy are presented as follows: the values of intensity of the signal from protons in various chemical bonds and states (which provides information about the proportional contents of nuclei in various chemical states in the sample under study) are plotted on the Y-axis; the values of the chemical shift of the proton signal relative to the reference zero, the signal from tetramethylsilane (SiC4H12) (which provides information about the number of different chemical compounds), are plotted on the X-axis.
Values of the chemical shift are expressed in parts per million (ppm) of the base frequency.
Findings
The results of the analysis of the chloroform extract from the Manchurian walnut mesocarp (a) and the juglone (b), which was obtained synthetically and dissolved in deuterated chloroform, are presented in the form of the spectra recorded on a 300 MHz NMR spectrometer (Bruker) (Fig. 2).
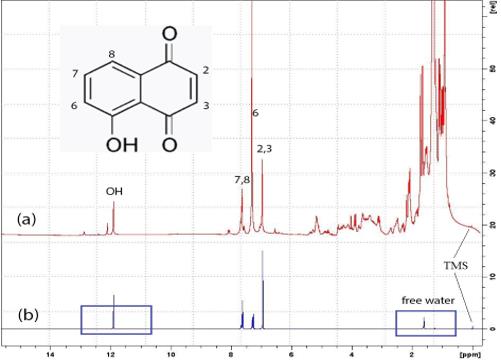
In the proton spectrum of 300 MHz, juglone has four peaks. Signals from 7 and 8, as well as from 2 and 3 CH-groups, at this resolution, merge into one peak. The signal from proton 6 merges with the signal of protonated chloroform (CHCl3); the higher level of the latter in the chloroform extract from the Manchurian walnut mesocarp is due to the residual chloroform after drying.
In our experiment, several signals appear in the proton NMR spectrum of the Manchurian walnut mesocarp extract (Fig. 2, a), as well as in the spectrum of synthesized juglone (Fig. 2, b). If only one form of the juglone molecule had been present in the analyzed sample, then only 1 peak at 11.89 ppm would be distinguished in the proton NMR spectrum.
The extract from the Manchurian walnut mesocarp is expected to contain a greater amount of substances than the synthesized juglone and its forms. The synthesized juglone should ideally contain only one form. However, a 15-fold magnification in the spectral range from 11.5 to 12.5 (Fig. 3) shows that the presence of other forms of 5-hydroxy-1,4-naphthoquinone is recorded but at low concentrations.
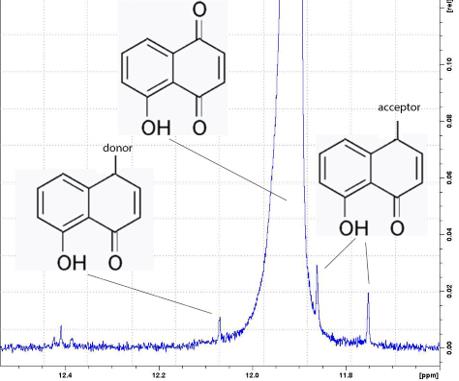
The content of all impurities is not higher than 0.1%, and, therefore, identifying their exact structure poses quite a serious challenge. However, it is possible to predict the presence of the expected forms of naphthoquinones in the studied mixture.
If oxygen is substituted by an acceptor, the signal from the hydroxyl group will shift to a weak field (leftward), if by the donor, to a strong field (rightward) (Fig. 3). The forms of acceptors and donors can be very different, and the presence of one or the other form depends on the type of synthesis of the molecule. 15-fold magnification of the spectrum in a range of 1.50–3.75 ppm (Fig. 4) shows that the studied mixture contains water in two forms: free and bound (in salts). The presence of these signals allows a conclusion that salts are one of the major impurities in the sample of synthetic juglone.
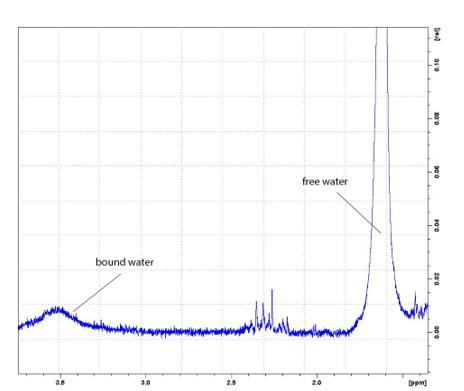
Conclusion
As the example of the studied naphthoquinones has shown, the synthetic and natural juglones are completely identical, which is confirmed by the similarity of their NMR signals in the spectra. Although some differences in the contents of impurities have been revealed, these contents are insignificant, and drawing any general conclusions about their effect on the biological activity of this medicinal agent is untimely.
Naphthoquinones have a very high biological activity, and even very low concentrations of various forms of naphthoquinones can exert significant effects on the vital activity of living organisms.
To make a definite conclusion about the biological activity of a particular form of synthetic naphthoquinones, all the accompanying forms of naphthoquinones contained, in addition to the base molecule, should be studied more in detail. This applies to any other synthesized and natural biomolecules.
References
Brasseur, L., Hennebert, E., Fievez, L., Caulier, G., Bureau, F., Tafforeau, L., Flammang, P., Gerbaux, P., & Eeckhaut, I. (2017). The roles of spinochromes in four shallow water tropical sea urchins and their potential as bioactive pharmacological agents. Marine Drugs, 15(6), 179.
Dayronas, Zh. V., & Zilfikarov, I. N. (2011). Natural naphthoquinones: prospects of medical application. MO, Shchyolkovo: Publisher Markhotin P. Yu.
Drozdov, A. L., Artyukov, A. A., & Elkin, Yu. N. (2017) Pigments in egg cells and epidermis of Sand Dollar. Scaphechinus mirabilis. Russian Journal of Developmental Biology, 48(4), 257-262.
Drozdov, K. A., Artyukov, A. A., & Drozdov A. L. (2021). Changes in the Composition of Celomic Fluid Metabolites of the Black Sea Urchin Mesocentrotus nudus (Echinoidea) and the Starfish Asterina pectinifera (Asteroidea) under Conditions of Hypoxia Stress. Biology Bulletin, 48(4), 407-412.
Dyshlovoy, S. A., Pelageev, D. N., Hauschild, J., Borisova, K. L., Kaune, M., Krisp, C., Venz, S., Sabutski, Y. E., Khmelevskaya, E. A., Busenbender, T., Denisenko, V. A.., Pokhilo, N. D., Atopkina, L. N., Graefen, M., Schlueter, H., Stonik, V. A., Bokemeyer, C., Anufriev, V. Ph., & von Amsberg, G. (2019). Successful targeting of the Warburg effect in prostate cancer by glucose-conjugated 1, 4-naphthoquinones. Cancers, 11(11), 1690, DOI: 10.3390/cancers11111690
Fedoreyev, S. A., Krylova, N. V., Mishchenko, N. P., Vasileva, E. A., Pislyagin, E. A., Iunikhina, O. V., Lavrov, V. F., Svitich, O. A., Ebralidze, L. K., & Leonova, G. N. (2018). Antiviral and antioxidant properties of Echinochrome A. Marine Drugs, 16(12), 509. DOI:
Hou, Y., Vasileva, E. A., Carne, A., McConnell, M., El-Din, A. B. A., & Mishchenko, N. P. (2018). Naphthoquinones of the spinochrome class: occurrence, isolation, biosynthesis and biomedical applications. RSC Advances, 8, 32637. DOI:
Hughes, W., Leoung, G., Kramer, F., Bozette, S. A., Safrin, S., Frame, P., Clumeck, N., Masur, H., Lancaster D., Chan, C. Lavellie, J., Rosenstock, J., Falloon, J., Feinberg, J., Lafon, S., & Rogers, M. (1993). Comparison of atovaquone (566C80) with trimethoprim-sulfamethoxazole to treat Pneumocystis carinii pneumonia in patients with AIDS. The New England Journal of Medicine, 328(21), 1521-1527.
Mishchenko, N. P., Krylova, N. V., Iunikhina, O. V., Vasileva, E. A., Likhatskaya, G. N., Pislyagin, E. A., Tarbeeva, D. V., Dmitrenok, P. S., & Fedoreyev, S. A. (2020). Antiviral potential of sea urchin aminated spinochromes against herpes simplex virus type 1. Marine Drugs, 18(11), 550,
Mishchenko, N. P.; Fedoreev, S. A., & Dogadova, L. P. (2004). Preparat gistokhrom dlya oftal'mologii [Histochrome in ophthalmology]. Vestnik DVO RAN, 3, 111-119.
Polonik, S. G., Prokofiev, N. G., Agafonova, I. G., & Uvarova, N. I. (2003). Antitumor and immunostimulating activity of O-, and S-acetylglucoside of 5-hydroxy-1, 4-naphthoquinone (juglone). Pharmaceutical Chemistry Journal, 37(8), 3-4.
Polonik, S. G., Tolkach, A. M., Shentsova, E. B., & Uvarova, N. I. (1995). Synthesis and cytostatic activity 2-bromine-3-alkiljugloneov and related thioglucoside on their basis. Pharmaceutical Chemistry Journal, 29(10), 668-671.
Roth, L. M., & Stay, B. (1958). The occurrence of para-quinones in some arthropods, with emphasis on the quinone-secreting tracheal glands of Diploptera punctata (Blattaria). Journal of Insect Physiology, 1, 305-318.
Sabutski, Y. E, Menchinskaya, E. S., Shevchenko, L. S., Chingizova, E. A., Chingizov, A. R., Popov, R. S., Denisenko, V. A., Mikhailov, V. V., Aminin, D. L., & Polonik, S. G. (2020). Synthesis and Evaluation of Antimicrobial and Cytotoxic Activity of Oxathiine-Fused Quinone-Thioglucoside Conjugates of Substituted 1,4-Naphthoquinones. Molecules, 25(16), 3577. DOI:
Vasileva, E. A., Mishchenko, N. P., Tran, V. T. T., Hieu, M. N., Vo H. M. N., & Fedoreyev, S. A. (2021). Spinochrome Identification and Quantification in Pacific Sea Urchin Shells, Coelomic Fluid and Eggs Using HPLC-DAD-MS. Marine Drugs, 1(19), 21. DOI:
Vo, H. M. N., Tran, V. T. T., Cao, H. T. T., Mischenko, N. P., Fedoreyev, S. A., & Truong, B. H. (2020). Polyhydroxynaphthoquinone pigment from Vietnam sea urchins as a potential bioactive ingredient in cosmeceuticals. Natural Product Communications, 15(11), 1-8.
Zhungietu, G. I., & Vlad, L. A. (1978). Yuglon i rodstvennyye 1, 4 naftokhinony [Juglone and related 1,4-naphtoquinonas]. Shtintsa.
Copyright information
This work is licensed under a Creative Commons Attribution-NonCommercial-NoDerivatives 4.0 International License.
About this article
Publication Date
03 June 2022
Article Doi
eBook ISBN
978-1-80296-125-6
Publisher
European Publisher
Volume
126
Print ISBN (optional)
-
Edition Number
1st Edition
Pages
1-1145
Subjects
Social sciences, education and psychology, technology and education, economics and law, interdisciplinary sciences
Cite this article as:
Drozdov, K. А., Sabutski, Y. E., Vshivkova, T. S., & Makarenko, V. P. (2022). Evaluation Of Differences Between Extracted And Synthesized Compounds On The Juglone Example. In N. G. Bogachenko (Ed.), AmurCon 2021: International Scientific Conference, vol 126. European Proceedings of Social and Behavioural Sciences (pp. 245-252). European Publisher. https://doi.org/10.15405/epsbs.2022.06.28